Evaluating Acrylamide Toxicokinetics in an In Vitro Integrated Organ Platform
James M. McKim1, David Austin1, Robert L. Sprando2, William Mattes2 and Suzanne Fitzpatrick2
1LifeNet Health LifeSciences, Kalamazoo, MI 49008, 2Food and Drug Administration, Center for Food Safety and Applied Nutrition, College Park Maryland
Abstract
The formation of Acrylamide (AA) in certain food types can occur when cooking at high temperatures. The formation of AA as well as other chemicals during high temperature food preparation and the subsequent formation of reactive metabolites have prompted regulatory agencies globally to investigate risk to human health. Given the large number of reactive chemicals formed during high temperature cooking and the need to develop human toxicokinetic and organ toxicity data for these compounds, alternative methods may provide a faster and less expensive means of performing initial assessments. Cell and tissue models today are more sophisticated in both their morphology and function. The use of these enhanced cell and tissue models in combination with new in vitro platforms is beginning to show promise for the eventual replacement of animal testing. The aim of this study was to test the ability of a novel Human Dynamic Multiple Organ Plate to provide data for key ADME parameters and organ toxicity using AA as the model compound. A three compartment (Intestine-Liver-Kidney) circuit was established. The intestine was EpiIntestinal from MatTek, Corp, human hepatocytes were from LifeNet Health LifeSciences, and Kidney cells (HK-2) were from ATCC. Each organ compartment was linked by a simulated blood system. The tubing of the blood system inside the organ compartment consisted of a semipermeable membrane. The movement of the test compound and its metabolites from the point of application to the other organ compartments was by osmotic diffusion into the simulated blood system. The simulated blood circulation was achieved with a micro syringe pump. Each organ compartment contained culture medium optimal for that organ’s growth and there was no net change in compartment fluid volumes.
To begin the study, aliquots of an AA stock (300 mg/mL) were diluted with medium to provide final exposure concentrations of 3, 5, and 10 mg in a final volume of 100 µL. This was then applied to the apical surface of the MatTek EpiIntestinal model. Samples from each organ compartment and from simulated blood were collected at 0, 5, 15, 30, 60, 90, 120, 240, 480, 1440 (24 hr), 2880 (48hr), and 4320 min (72 hr). AA movement was measured using LC/MS/MS. At 72 hr samples were collected for determining cytotoxicity. AA was detectable in the intestinal compartment at 30 min, reaching a maximum at 1.5-2 hr. Values returned to baseline after 24-48 hr. In liver and kidney, AA was detected at 1-1.5 hr reaching a maximum after 20-24 hr. AA concentrations were dose and time dependent in each compartment. After 72 hr of exposure to 3 mg AA, intestinal viability was 70%, while the 10 mg dose resulted in a viability less than 60%. Liver ATP and GSH decreased in a dose-dependent manner after 72hr. In kidney, NAG and KIM-1 release also followed a dose-dependent pattern. Analysis of kinetic data enabled key ADME parameters (Cmax, AUC, and T1/2) to be estimated. In conclusion, the novel Human Dynamic Multiple Organ Plate system predicted AA liver and kidney toxicity and provided key ADME data points. These findings indicate the system can be used as a rapid response model for ADME and toxicity, as well as a model to explore metabolites and mechanisms of adverse effects.
Introduction
Microphysiological Systems (MPS) are in vitro models that comprise multiple organ models (cells, 3D tissues, spheroids) interconnected by simulated blood flow. In recent years several iterations of MPS systems have appeared each with unique advantages and also with limitations. The ability to interconnect physiologically relevant organ systems offers the possibility of using in vitro testing to predict in vivo responses. Pharmacokinetic data combined with metabolism and organ specific safety data would enable the development of physiologically based pharmacokinetic models (PBPK) which in turn would provide much stronger and more reliable in vitro to in vivo extrapolations. The aim of this study was to evaluate a novel human dynamic integrated organ platform (Intestine-Liver-Kidney) with chemicals of interest to the US FDA by studying kinetic movement, metabolism, and cytotoxicity.
Materials and Methods
Preparation of Plates. A three-organ plate system (Intestine-Liver-Kidney circuit) (Figure 1) was used and equipped with a simulated blood system that consisted of tubing connected to a semipermeable membrane. The section of semipermeable membrane was 3 cm in length. The tubing was custom fit into the plate, such that only the dialysis membrane was in contact with each organ compartment. A perfusion rate of 5 μl/min was used in each experiment.
Intestinal Compartment. The EpiIntestinal™ 3D human tissue from MatTek, Corp. was used for the intestine chamber. Tissues were cultured under standard conditions on transwell inserts. Tight junctions were assessed by transepithelial electrical resistance (TEER). The EpiIntestinal™ tissues were placed into the plate systems (Figure 1) and connected to the liver compartment via simulated blood system (Figure 2).
Liver Compartment. The liver compartment consisted of fully characterized primary human hepatocytes (LifeNet Health) cultured on collagen coated wells. Cells were seeded in the system culture cups in culture media at a density of 500,000 cells/well and incubated at 37oC, 5% CO2 for 48 hr prior to beginning the experiments.
Kidney Compartment. The kidney compartment consisted of a human renal cell line (HK-2) from ATCC. The cells were added to the culture cup in culture media at a density of 1.1 x 106 cells/well and incubated at 37oC, 5% CO2 for 24 hr prior to beginning the experiments.
Dosing Regimen. After equilibration, the test material was added to the apical side of the intestinal chamber to simulate an oral exposure at time 0. Acrylamide was administered as an applied doses of (0.3, 0.5, and 1.0 mg total mass) in a volume of 100 μL from stock solutions of 3, 5 and 10 mg/mL.
Analytical Procedures. Acrylamide was monitored by LC-MS/MS. Standard curves and QC samples were prepared in PBS and compared to standard curves and QC samples in media with and without serum.
Results
Figure 1. LifeNet Health's Novel Human Dynamic Multiple Organ System
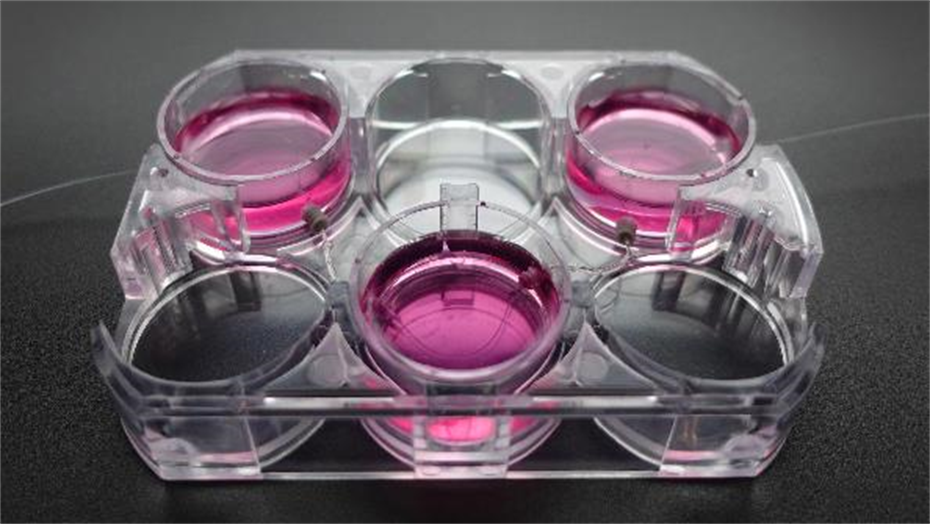
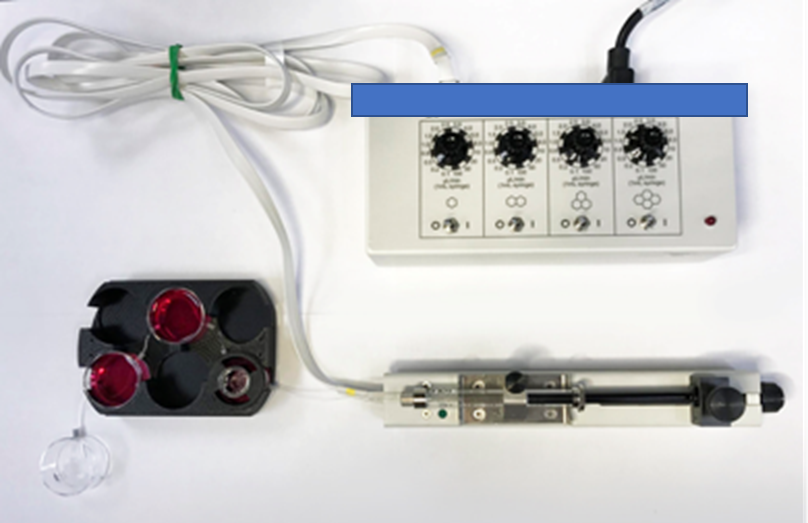
Figure 2. Human Dynamic Multiple Organ System with Three Organs Connected by a Simulated Blood System.
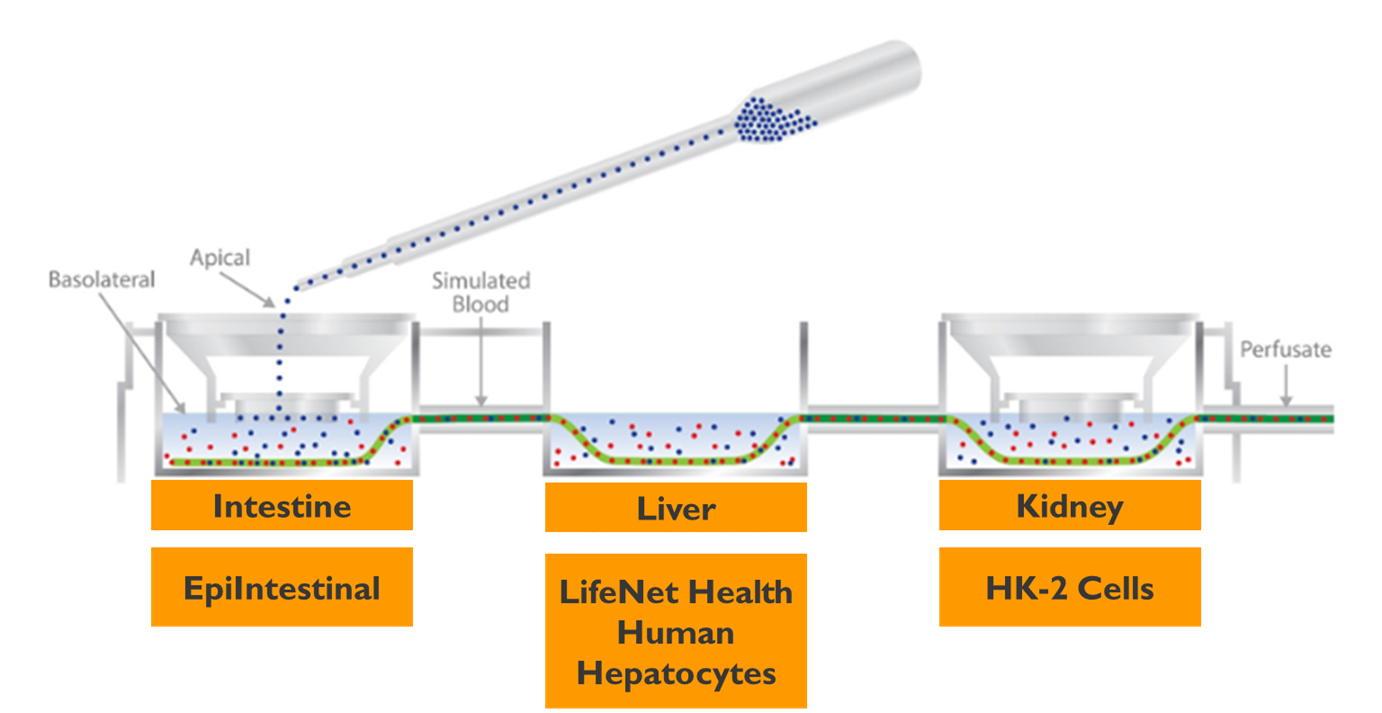
Figure 3. Acrylamide Kinetic Data for Intestine, Liver, and Kidney Chambers.
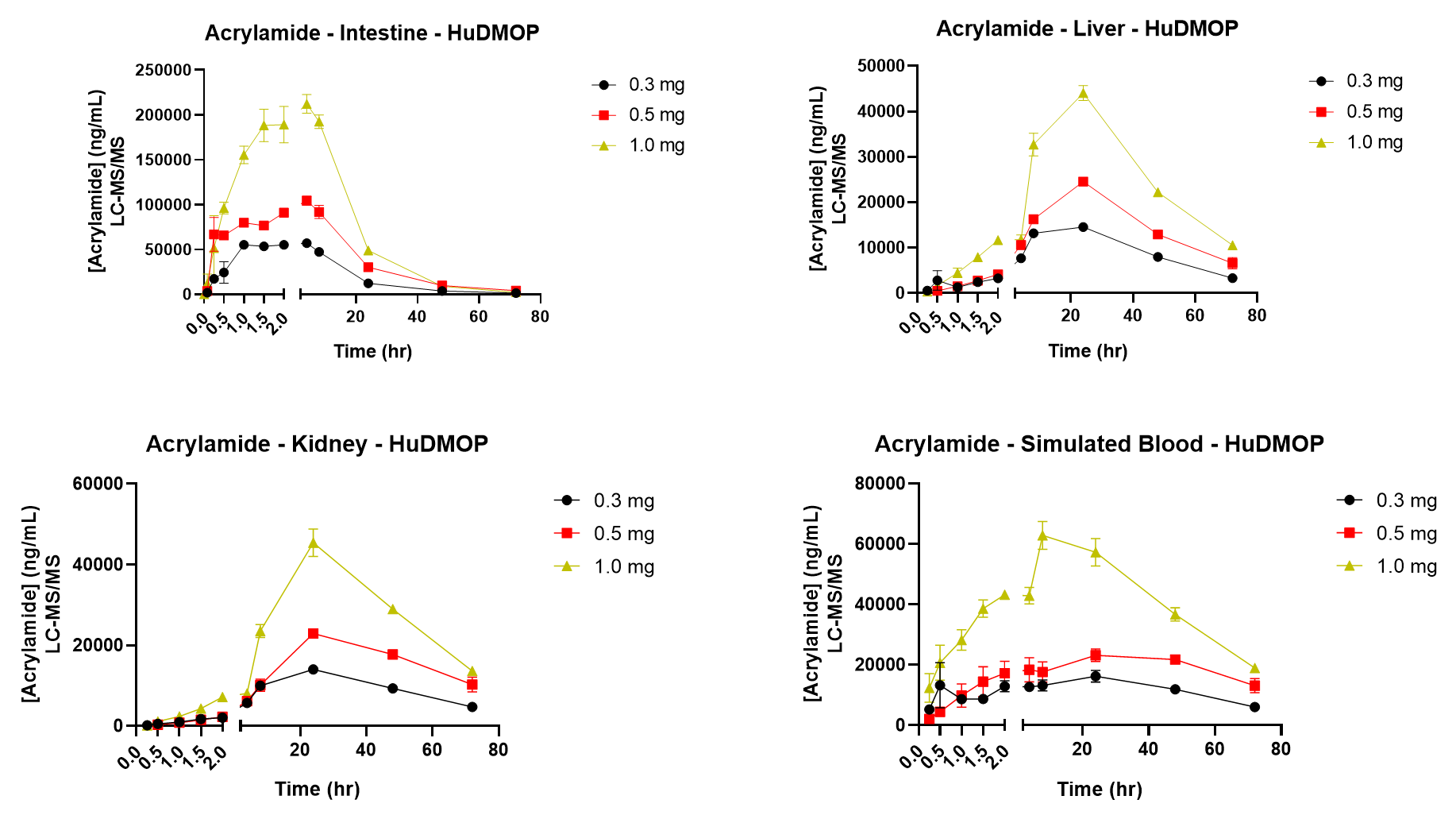
Figure 4. Acrylamide Intestinal Permeability
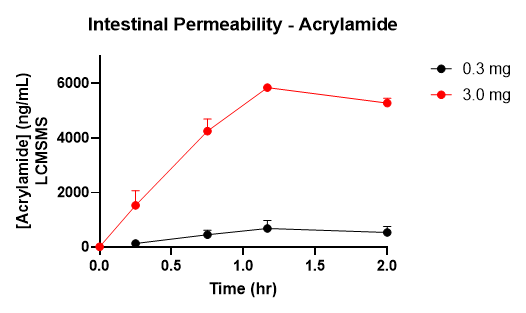
Figure 5. Effects of Acrylamide on Glutathione Levels
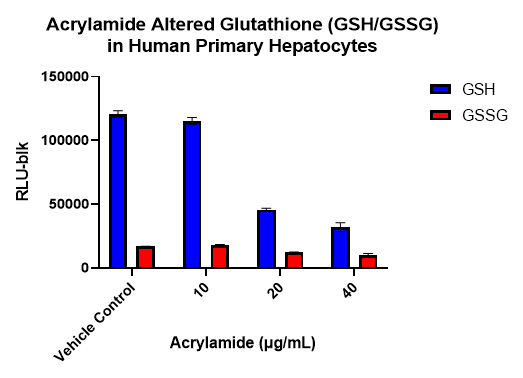
Figure 6. Effects of Acrylamide in the Integrated Organ System on Intestine, Liver, and Kidney Cell Health
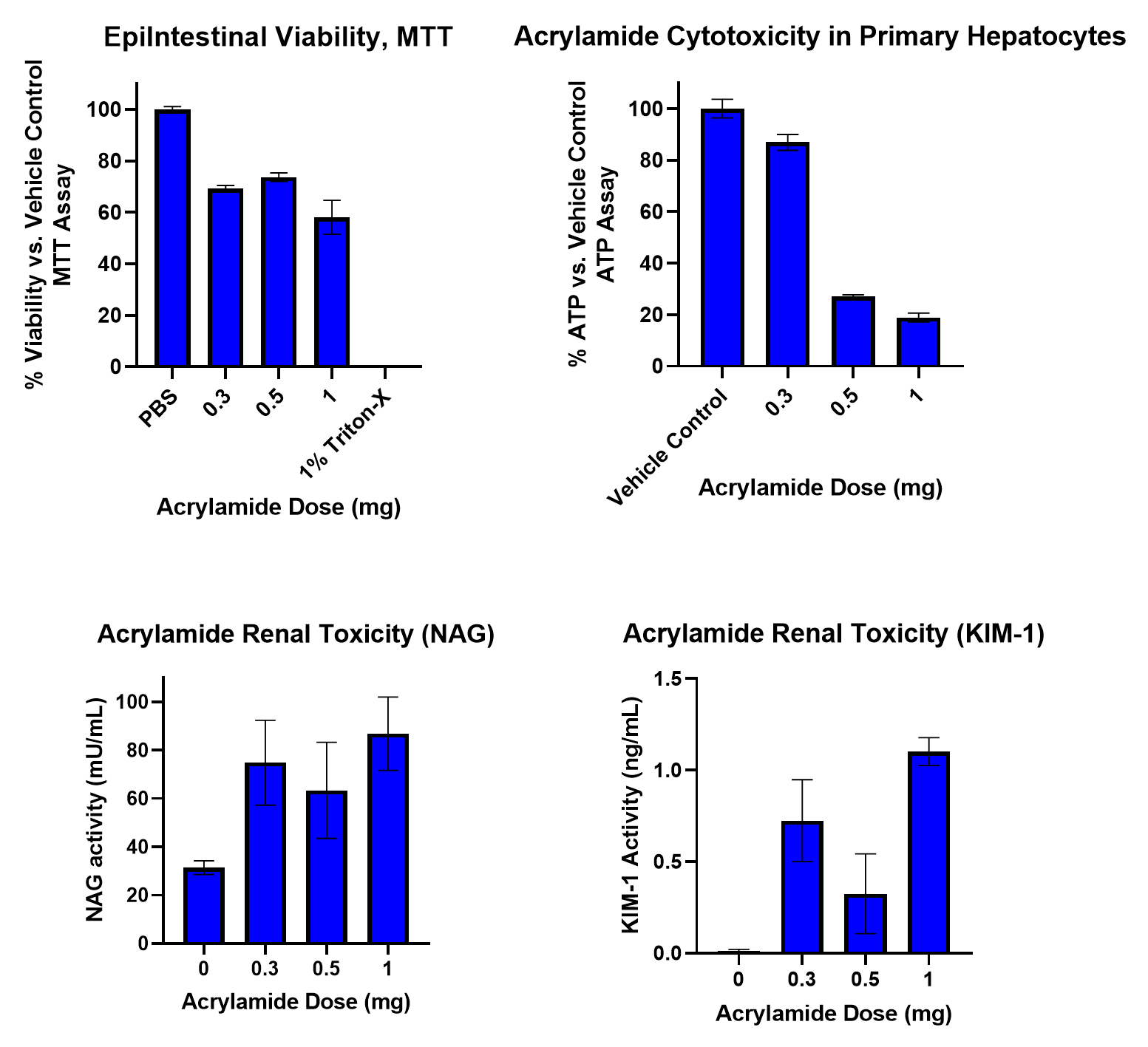
Conclusion
The novel in vitro human dynamic integrated organ platform (Intestine-Liver-Kidney) described provided pharmacokinetic data and cytotoxicity data that is in close agreement with those reported in the published literature.
There was rapid and efficient uptake of Acrylamide from the intestine.
Organ specific toxicity (liver and kidney) was identified.
The novel system demonstrated an ability to determine kinetic parameters, and some ADME endpoints. These findings indicate that the system described could be used to develop PBPK models to assist in vitro to in vivo extrapolation.
References/Acknowledgements
Fuhr, U., et al. (2006) Toxicokinetics of acrylamide in humans after ingestion of a defined dose in a test meal to improve risk assessment for acrylamide. Cancer Epid Biomarkers 15, 266-271.
Wikswo,J. (2014) The relevance and potential roles of microphysiological systems in biology and medicine. Exp Biol Med 239, 1061-1072.