Cryopreserved Primary Human Thyrocytes for Discovery and Development of Thyroid Disease Treatments
E. Rogers1, T. Nguyen-Jones1, K.K. Wolf2, T. Stone2, E.L. LeCluyse2
Introduction
Thyroid disease is a growing health concern, and more than 12 percent of the U.S. population will experience a thyroid condition at some point in their lives with many cases going undiagnosed. Besides iodine deficiency that is considered the main cause for hypothyroidism, chemical exposure is another contributing factor of thyroid dysfunction. For example, increases and decreases in thyroid hormone synthesis can be caused by chemicals that are agonists or antagonists of the thyroid stimulating hormone receptor (TSHR).1 Animal or cell line models are common test models for thyroid disrupting compounds. However, these test models are far from being accurate representatives of human receptor biology and their corresponding signaling pathways that lead to changes in thyroid hormone production. In addition, animal-testing is costly and time consuming while cell lines often lose their original characteristics after multiple passages or transformation. As such, the field needs a physiologically relevant human thyroid model that mimics normal endocrine biology, which will allow for prioritization of TDCs and the reduction of animal testing in discovery and development of new compounds. To address this problem, the U.S. Environmental Protection Agency (US EPA) has developed a 3D human thyrocyte microtissue assay to screen for chemical molecules that could potentially lead to disruptions of thyroid receptor function in humans.2 However, only partial reductions in T4 production were observed using a known TSHR antagonist under the culture conditions used in the study. The aim of this study was to develop a novel 2D human thyroid model system for TSHR active compounds using cryopreserved primary human thyroid epithelial cells and optimize assay conditions for screening purposes, including seeding density, length of culture period with thyroid stimulating hormone receptor (TSHR) activators and compound exposure period for testing. Thyrocytes were cultured in a standard 96-well plate format and the effect of TSH exposure period on target gene expression was investigated. The concentration-response profiles were determined for native (hTSH, bTSH) and antibody-based (M22, Ki18) thyroid stimulating hormone receptor (TSHR) activators. In addition, the inhibitory effect of a TSHR antagonist (Ki70) on M22-induced target gene expression was also evaluated using two different approaches: therapeutic and preventive treatment.
Materials & Methods
Cell Culture of Cryopreserved Thyrocytes
Thyrocytes from healthy donors were isolated as described previously2. The cryopreserved thyrocytes (p1) were thawed in h7H base human thyrocyte plating media (HTPM) and plated in 96-well TC-treated culture plates. Cell cultures were treated with native TSHR activators (bovine and human) or antibody-based activators (M22 IgG (+) and K1-18 IgG (+))
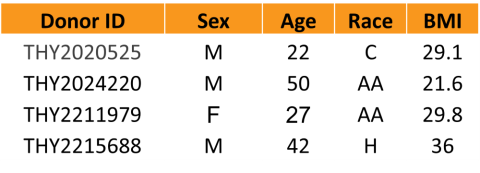
TG Inhibitory Study
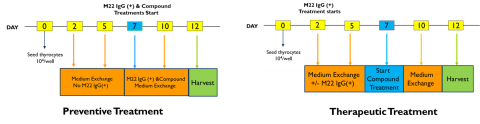
Assay Analysis
For mRNA analysis, total RNA was isolated using Qiagen RNEasy Mini Kit (Qiagen, 74106) and quantified with NanoDrop 8000 spectrophotometer (ThermoFisher, ND-8000-GL). Isolated total mRNA was reverse transcribed into cDNA using the High- Capacity cDNA Reverse Transcription Kit (Applied Biosystem, 4368813). qPCR analysis was performed using Tagman Universal PCR Mastermix (Applied Biosystem, 4304437) with the following probe (ThermoFisher): Hs00427620_m1 (TBP) and hs00794359_m1 (TG).
TG production at protein level was analyzed using ELISA kits (Invitrogen, EHTG).
Data Analysis
Data were normalized to vehicle controls and plotted using GraphPad Prism.
Results

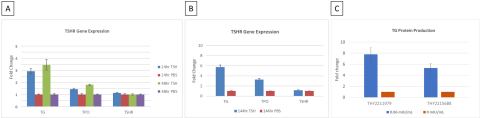
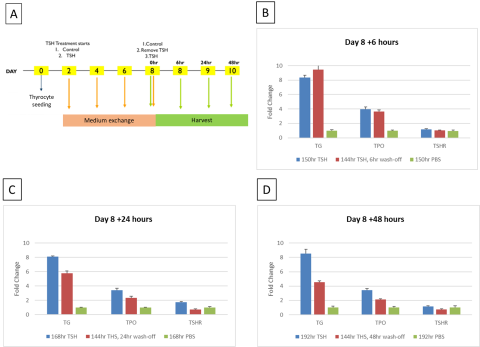
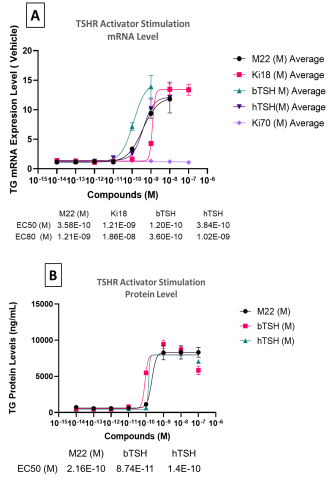
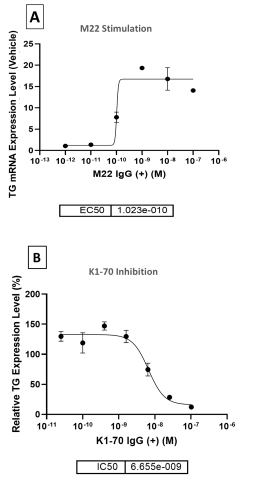
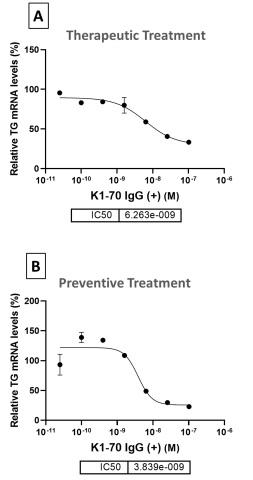
Summary & Conclusions
- TG was identified as the reporter gene for thyrocyte model validation with the time of exposure to the activators of 8 days (n=3).
- TG mRNA and protein levels were upregulated by TSHR activators in a dose-dependent manner while TSHR antagonist, K1-70, inhibited TG production by >80%.
- Preventative treatment using K1-70 to antagonize M22 antibody activation of TSHR resulted in a more robust inhibition response compared to therapeutic treatment.
- The 2D primary thyrocyte model system was able to reproduce autoimmune thyroid disease (AITD) response using TSHR activators.
- Cryopreserved primary human thyrocytes in a standard 2D model represents a promising, simpler new tool for screening TSHR active compounds.
References/Acknowledgements
1. Crofton, K. (2008) Thyroid disrupting chemicals: mechanisms and mixtures. Int. J. Androl 31: 209-223
2. Deisenroth C et al. (2019) Development of an in vitro human thyroid microtissue model for chemical screening. Toxicol Sci 174: 63-78